Versatile Switch Mode Power Supply PWM Controller [ 150100 ]
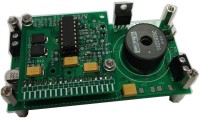
Although SMPS (switch mode power supplies) can take various structures (Buck, Boost, Flyback, Forward, etc.), they are always based o
Although SMPS (switch mode power supplies) can take various structures (Buck, Boost, Flyback, Forward, etc.), they are always based on a power stage and control block. With this in mind I decided to design a configurable generic control card depending on the type of power supply to implement.
(English version translated with https://translate.google.fr, if my english is too bad send me a post in order to correct my mistake, French version at the bottom)
Introduction
PWM generic card is designed in order to implement different kind of smps (swtich mode power supplies) with flexibility, reducing design and manufacturing costs through the use of a single development platform. This ambitious project, but nevertheless feasible requires early to set targets, by writing the specifications.
Notes : The theoretical description of switching power supplies beyond the scope of this article and is not reviewed, more about this is adequately covered on the Web and books [1], [2] and [3] for the reader can get the basic knowledge required to understand the different paragraphs.
Specifications
The generic control card can be used on most isolated topologies
uninsulated (Buck, Boost, Buck-Boost, Flyback, Forward, Push-Pull, Half-Bridge, Full-Bridge and derivatives). The most basic functions used in switching power supply must be featured on this card, these functions are listed below.
- Compatible with Current-mode, Voltage-mode and Voltage-mode with a Feed-Forward loop.
- Current limiting stage of the power supply output.
- Adjustable switching frequency.
- Error amplifier compatible with 1, 2, 3 type or follower, see [1], [2] et [3].
- Configurable « Under Voltage Lockout » et « Over-Voltage Lockout ».
- Provide an adjustable « Soft-Start » system.
This specification is synthesized by the functional diagram of Figure 1 (see Figures.zip).
Design
The design of the card is based primarily on the needs expressed
preceding paragraph and the manufacturer's documentation [4], [5], [6] and [7].
Each functional block of Figure 1 is identified in the diagram (see Figure 8), which allows to design function by function.
Controller
The first step is to select a controller type, from my personal experience I immediately selected the UC2825 controller family (UC2823, UC2824, UC2825 or UC28025) from TI (Texas Instruments).
This type of controller is compatible with most power topologies (with some adjustments of the power section). However this choice adds two requirements, the first is to limit the input voltage between 10V and 30V and the second is to add a protection circuit against transients on the outputs of the PWM (TI recommendations).
Decoupling of power supplies
Decoupling of each power supply is provided by two different kinds capacitors of in parallel (1 ceramics for filtering RF noise 1 tantalum or chemical for energy storage).
These capacitors are C1, C5 for "signal" supply , C2, C6 for the reference voltage provided by the controller MA1, C3, C7 to the power supply and C4, C8 to the external reference voltage.
Finally SJ1 and SJ2 allow either isolate or connect the "power" supply and "signal".
Switching Frequency
The switching frequency of the controller is set by the R9 resistor and C13 capacitor.
These values are estimated based on the desired switching frequency and the desired time-out, based on the controller datasheets [4], [5], [6] and [7].
Note: These items also adjust the period and time to the high state of the signal CLK '.
Current Limitation & Shut-down # 1
The current limiting is achieved by abortion commands cycles
PWM outputs. This circuit consists of the R21 and R23resistors which form a voltage divider and C15 capacitor, these elements allow to adapt and filter the image voltage current limit or the sleep command.
Output Protection
This circuit is based on a preconceived block, based on the TI recommendations [4], [5], [6] and [7]. The outputs must be capable of driving MOS transistors, it is necessary to protect them from possible "undershoot / overshoot" due to parasitic capacitances of the transistors.
This protection is provided by two clipping diodes on each output (D1, D2 on the output B and D3, D4 on the output A). These outputs should also impose a state of rest to the transistors in the controller's startup phase.
This transistor state is imposed either by a pull-up resistor (R6, R12) or pull-down (R8, R13).
Voltage Reference
The reference voltage of the error amplifier is obtained on R10 terminals, by dividing the internal reference voltage of the controller if the R5 resistor is wired, or external reference if the R4 resistor is mounted. These values are depending on the application (voltage, accuracy, etc.).
Shut-down # 2
The Standby mode can also be provided by this circuit, by shorting
the entry "soft-start" of the controller to ground via the Q2 transistor. The R18 and R19 resistors are used to adapt the 5V to the Q2 transistor. The D6 diode performs a "or logical" with D5, between "Shut-down # 2" and "Under-Voltage Lockout" functions.
Under-Voltage Lockout
This function enables the circuit of "Shut-down # 2" function via the D5 diode when the
Q1 transistor is on. And the controller is maintained in standby, until the voltage on the base of Q1 transistor is less than the VREF voltage (5V or approximately). The threshold circuit "Under-Voltage Lockout" is configurable by the R15 and R17 resistors.
Over-Voltage Lockout
This function is a copy of the "Shut-down #2" function, the Q5 transistor by shorting the input "soft-start" to the ground controller. As long as the Q5 transistor is on, the controller is maintained in standby. The trigger threshold is configurable by the R28 and R29 resistors.
Soft-start
The soft start controller is set by the capacitor C12. The value of this capacity is estimated based on the application requirements and the datasheets [4], [5], [6] and [7] or formula below.
C12 = Ichargemin * Tstartmin / EAoutmin
C12 ≈ 3uA * Tstartmin / 0.5V
Where Ichargemin and EAoutmin are from the datasheets, Tstartmin represents the desired soft-start period.
Ramp generator
The basic principle of a PWM signal is to modulate a pulse width, by
a comparison between the sawtooth signal and an error signal (the result of the difference between a reference voltage and an image of the output voltage to be regulated). This principle is shown in Figure 2 and illustrated in Figure 3, for different values of the error signal.
In conclusion, the generation of a sawtooth signal on pin 'ramp' the controller, is essential to the operation of the PWM (see datasheets [4], [5], [6] or [7] ).
This type of signal can be generated in different ways according to that one uses a so-called voltage regulation "simple", a current control or voltage regulation with feedforward loop called "Feed-Forward".
Voltage mode
The ramp generator for the voltage mode is provided by R14 resistor. This 0Ω resistance ensures the looping between pins 'Ct' and 'Ramp' of the controller, see the datasheets [4], [5], [6] and [7].
Current mode
The ramp signal for current mode is taken from the outlet of the filter formed by R16 resistor and C14 capacitor, see the datasheets [4], [5], [6] and [7].
Voltage mode with "Feed-Forward"
This control method requires generating a periodic saw-tooth signal, whose amplitude changes as a function of the supply voltage, as shown in Figure 4 (for more details see [2], [8] and [9 ]).
The generation of the ramp signal is provided by the schematic in Figure 5 designed on the principle scheme proposed by the manufacturer (see the datasheets [4], [5], [6] or [7]) and exposed theories by documents [8] and [9].
The reading (from left to right) of the schematic of the Figure 5 (the principle is illustrated in Figure 6) shows that the ramp signal is generated by the C17 capacitor, which is charged through R20 resistor and discharged by R24 resistor when Q3 transistor is saturated. This transistor is controlled by the CLK signal, whose the DC component (about 2.3V) is suppressed by the filter composed of R26 resistor and C16 capacitor. However, depending on the value of C16 capacitor, the CLK signal needs to be amplified in power, which is achieved by the follower circuit (Q4 transistor and R22 and R25 resistors). The Q4 transistor also ensures a role in C16 discharge, when the CLK signal returns low. Finally, the amplitude of the ramp is limited to about 6V by the D7 diode.
The R27 resistor (0Ω) offers the possibility to isolate the structure to facilitate the tuning.
The component values are estimated as follows.
At first the values of R24 and C17 should allow to completely discharge the capacitor during the high state of the CLK signal, either during the timeout period 'td'. This requirement imposes the criterion below.
R24 * C17= td / 20
Consequently
C17≤ (td / 20) * R24
Then, the previously calculated value of C17 allows to estimate the R20 resistor, as a function of the voltage VFF, the desired voltage RAMP and switching periods TCLK and dead time 'td'.
R20= VFF / (20 * Irampe)
With
Irampe=( Vrampepk * C17) / (TCLK - td)
Finally, the C16 capacitor and the R26 resistance are calculated to ensure the conduction of Q3 transistor during the 'td' timeout period. These elements are estimated based on the following equations.
C16 ≤ 1e-3 * td
R26 * C16 = 5 * td
Test Points
The test points allow to do any measurements of the controller signals without disrupting them.
Error amplifier / corrector
This function is probably the most important because it is the key to proper regulation. This circuit is based on a preconceived block can be configured as error amplifier either type 1, 2, 3 or follower according to the needs of the application.
The study and design of the error amplifier is based on the books [1] or [2].
The different configurations are as follows.
- If the internal amplifier of the controller is used as a follower, in this case the R2 and R11 resistors are connected, C10 capacitor replaced with 0Ω resistance and value of the R7 resistor is adjusted according to the need.
- If the internal amplifier is used in the controller as error amplifier type 1, 2 or 3, then the R11 resistor is not mounted and the values of other elements depend on the type of error amplifier to implement, as well as estimates.
This paragraph concludes the card design, let's go to the practical realization.
Practical realization
The practical realization of such a card is not an easy task because it is based in major part on the experience of the designer, which is only acquired through practice, so I naturally started with the production of prototype on proto-board. These cards have allowed me to get into the development and verification of the feasibility of prototypes that I could modify by soldering. After three versions of prototype I got to the fourth version that was layouted, see the PCB 9 and 10. MA1 controller is mounted on a DIP16 support for easy replacement in case of damage.
J1 can be either be a straight or angled breakable strip.
After manufacturing the pcb, simply configure the various functions to start a first application.
Application
During the development of the first prototype boards, I realized two smps types, a buck and Forward (see photos 1, 2 & 3). So I of course re-use the power boards of these power supplies to complete the development and to do the first tests to compare the performance between the prototype boards pelleted and routed cards.
Over-Voltage LockOut improvement
At the time to complete this article, I added the improvement described below.
In effect, the basic design of the Over-Voltage Lockout circuit does not allow
program a precise threshold. This defect is primarily due to the variation of the Vbe voltage of the Q5 transistor. This value can vary between 0.5V and 1V, it follows that the threshold value, calculated using the equation below, can vary from simple to double (ie a 100% error).
SeuilOVLO = VbeQ5 * (R28+R29)/R29
This problem can be solved by the addition of the small circuit proposed in Figure 7. This scheme enables the Q5 transistor (ie the Over-Voltage Lockout system) when the Q6 transistor is on, when the voltage across the resistor R31 exceeds the value of VREF + VbeQ6 (between 5.6V and 6.1V approximately). This principle reduces the error of the threshold voltage of the Over-Voltage System Lockout value of 5.85V +/- 5%.
Thus, the threshold calculation becomes the following.
SeuilOVLO = (VREF + VbeQ6) * (R31+R32)/R31
For this, the component values are:
Q6 = MMBT2907 (SOT23)
R29 = 10k (0805)
R30 = 100k (0805)
R31 = 10k (0805)
R32 Resistor is calculated using the equation below and the desired threshold value.
R32 = [SeuilOVLO - (VREF + VbeQ6)] / [(VREF + VbeQ6)/R31]
Note: in case of the use of this circuit, R28 must be unconnected.
In conclusion this enhancement significantly reduces the circuit threshold error of Over-
Voltage Lockout and will be implemented in the next release of PCBs. Nonetheless photos 2 and 3 suggest included an example implementation, for those of you wishing to use this enhancement on version 04 of the PCB.
Conclusion
The results obtained with the pcb are very close to those obtained with prototyping boards, demonstrating, despite their reputation, that the discrete component switching power supplies are accessible even to hobbyists, provided that the wiring is neat and if one puts out the EMC aspect.
Link
[1] Switch-Mode Power Supplies Spice Simulations and Practical Designs, C Basso, 2010, ISBN-13 : 978-8183332910
[2] Switching Power Supply A to Z, S. Maniktala, 2006, ISBN-13 : 978-0750679701
[3] Power Supply Cookbook, Second Edition, M. Brown, 2001, ISBN-13 : 978-0750673297
[4] Datasheets UC2823 : http://www.ti.com/lit/ds/symlink/uc2823.pdf
[5] Datasheets UC2824 : http://www.ti.com/lit/ds/symlink/uc2824.pdf
[6] Datasheets UC2825 : http://www.ti.com/lit/ds/symlink/uc2825.pdf
[7] Datasheets UC28025 : http://www.ti.com/lit/ds/symlink/uc28025.pdf
[8] Datasheets CS51221 : http://www.onsemi.com/pub_link/Collateral/CS51221-D.pdf
[9] Datasheets LM25037 : http://www.ti.com/lit/ds/symlink/lm25037.pdf
============================================================
Version française
============================================================
Bien que les alimentations à découpage puissent se présenter sous différentes structures (Buck, Boost, Flyback, Forward, etc.), ces dernières reposent toujours sur un bloc puissance et un bloc commande. Partant de ce constat j’ai décidé de concevoir une carte commande générique configurable en fonction du type d’alimentation à mettre en œuvre.
Introduction
La carte générique PWM est conçue pour permettre la mise en œuvre des différente
différentes topologies d’alimentation à découpage avec un maximum de flexibilité, en réduisant les coûts d’étude et de fabrication par l’utilisation d’une
plateforme unique de développement. Ce projet ambitieux, mais néanmoins
réalisable nécessite dès le début de fixer les objectifs à atteindre, par la rédaction d’un petit cahier des charges.
Nota : La description théorique des alimentations à découpage sort du cadre de cet article et n’est donc pas revue, de plus ce sujet est suffisamment couvert sur le WEB et les ouvrages [1], [2] et [3], pour que le lecteur puisse obtenir les connaissances de base nécessaires à la compréhension des différents paragraphes.
Cahier des charges
La carte commande générique se veut utilisable sur la plupart des topologies isolées
et non isolées (Buck, Boost, Buck-Boost, Flyback, Forward, Push-Pull, Half-Bridge, Full-Bridge et dérivées). Cette carte doit donc embarquer la plupart les fonctions de base utilisées en matière d’alimentation à découpage, ces fonctions sont listées ci ci-après.
- Compatible des modes de régulation en tension et en courant.
- Proposer un mode de régulation en tension comportant une boucle de régulation par anticipation, dite « Feed-Forward » (nous reviendrons en détail sur cette fonction un peu plus tard).
- Disposer d’un étage de limitation du courant, de sortie de l’alimentation.
- Offrir la possibilité d’ajuster la fréquence de découpage.
- Permettre de configurer l’amplificateur d’erreur en type 1, 2, 3 ou suiveur, voir [1], [2] et [3].
- Comporter un système « Under Voltage Lockout » et « Over-Voltage Lockout » paramétrable.
- Proposer un système de « soft-start » à durée ajustable, assurant un démarrage progressif du PWM.
- Disposer d’une entrée permettant de mettre le contrôleur en mode veille.
Ce cahier des charges est synthétisé par le diagramme fonctionnel de la figure 1, à la base de la conception de la carte.
Conception
La conception de la carte repose principalement sur les exigences exprimées au
paragraphe précédent, ainsi que sur les documents constructeur [4], [5], [6] et [7].
Chacune des fonctions du diagramme fonctionnel de la figure 1 est identifiée sur le schéma en figure 8, ce qui permet de décomposer l’étude de la carte fonction par
fonction.
Contrôleur
La première étape consiste à sélectionner un type de contrôleur, par expérience personnelle, j’ai immédiatement retenu la famille de contrôleurs UC2823, UC2824, UC2825 ou UC28025 de chez TI (Texas Instruments).
Ce type de contrôleur est compatible de la plupart des topologies d’alimentation (moyennant quelques adaptations de la partie puissance). Cependant ce choix ajoute deux exigences, la première est de limiter la tension d’entrée entre 10V et 30V et la seconde est d’ajouter un circuit de protection contre les transitoires sur les sorties du PWM (recommandations TI).
Découplage des alimentations
Le découplage de chaque alimentation est assuré par deux condensateurs, de natures différentes en parallèle (1 céramique pour le filtrage du bruit HF, 1 tantale ou chimique comme réservoir d’énergie).
Ces condensateurs sont C1, C5 pour l’alimentation dite de « signal », C2, C6 pour la tension de référence fournie par le contrôleur MA1, C3, C7 pour l’alimentation de puissance et C4, C8 pour la tension de référence externe.
Enfin les ponts de soudures SJ1 et SJ2 permettent soit d’isoler, soit de relier les
alimentations de puissance et de « signal ».
Fréquence du contrôleur
La fréquence du contrôleur est fixée par la résistance R9 et le condensateur C13.
Les valeurs de ces composants sont estimées en fonction de la fréquence de découpage souhaitée et du temps mort désiré, en s'appuyant sur les abaques des datasheets du contrôleur [4], [5], [6] ou [7].
Nota : Ces éléments dimensionnent aussi la période et le temps à l’état haut du signal ‘CLK’.
Limitation du courant & Shut-down #1
La limitation du courant est réalisée par avortement des cycles de commandes des
sorties du PWM. Ce circuit est composé des résistances R21 et R23 qui forment un diviseur de tension et du condensateur C15, ces éléments permettent d'adapter et de filtrer la tension image du courant à limiter ou la commande de mise en veille.
Protection des sorties
Cet étage est basé sur un bloc préconçu, qui s'appuie sur les recommandations des
datasheets du contrôleur [4], [5], [6] et [7]. Les sorties étant susceptibles de piloter des transistors MOS, il est nécessaire de les protéger d'éventuelles "undershoot/overshoot" dues aux capacités parasites des transistors.
Cette protection est assurée par deux diodes d’écrêtage sur chaque sortie (D1, D2 sur la sortie B et D3, D4 sur la sortie A). Ces sorties doivent aussi imposer un état repos aux transistors lors de la phase de démarrage du contrôleur.
Cet état repos des transistors est imposé soit par une résistance de pull-up (R6, R12) ou pull-down (R8, R13) suivant la configuration.
Référence de tension
La référence de tension, de l'amplificateur d'erreur, est obtenue aux bornes de R10, par division de la tension de référence interne du contrôleur si la résistance R5 est câblée, ou de la référence externe si la résistance R4 est montée. Les valeurs de ces composants sont estimées en fonction de l'application (tension, précision, etc.).
Shut-down #2
La mise en veille de la carte peut aussi être assurée par ce circuit, en court-circuitant
l’entrée « soft-start » du contrôleur à la masse par l’intermédiaire du transistor Q2. Les résistances R18 et R19 servent à adapter la commande 5V au transistor Q2. La diode D6 réalise une fonction « ou logique » avec D5, entre les fonctions Shut-down #2 et Under-Voltage Lockout.
Under-Voltage LockOut
Cette fonction active le circuit de la fonction Shut-down #2, via la diode D5 lorsque le
transistor Q1 est passant. Ainsi le contrôleur est maintenu en veille, tant que la tension sur la base du transistor Q1 est inférieure à la tension VREF (soit 5V environ). Le seuil de déclenchement du circuit Under-Voltage LockOut est configurable par les résistances R15 et R17.
Over-Voltage LockOut
Cette fonction est une copie de la fonction Shut-down #2, le transistor Q5 court-circuite l’entrée « soft-start » du contrôleur à la masse. Ainsi le contrôleur est maintenu en veille, tant que le transistor Q5 est passant. Le seuil de déclenchement du circuit Over-Voltage LockOut est configurable par les résistances R28 et R29.
Soft-start
Le démarrage progressif du contrôleur est fixé par le condensateur C12. La valeur de cette capacité est estimée en fonction des exigences de l'application, en s'appuyant sur les datasheets du contrôleur [4], [5], [6] et [7] ou de la formule ci-dessous.
C12 = Ichargemin * Tstartmin / EAoutmin
C12 ≈ 3uA * Tstartmin / 0.5V
Où Ichargemin et EAoutmin sont issus des datasheets du contructeur et Tstartmin représente la période de démarrage progressif désirée.
Générateur de rampe
Le principe de base d'un PWM est de moduler un signal en largeur d’impulsion, par
comparaison entre un signal en dent de scie et un signal d'erreur (résultat de la différence entre une tension de référence et une image de la tension de sortie à réguler). Ce principe est présenté en figure 2 et illustré en figure 3, pour différentes valeurs du signal d'erreur.
En conclusion, la génération d'un signal en dent de scie, sur la broche 'ramp' du contrôleur, est indispensable au fonctionnement du PWM (voir les datasheets du contrôleur [4], [5], [6] ou [7]).
Ce type de signal peut être généré de différentes manières suivant que l'on utilise une régulation en tension dite "simple", une régulation en courant ou une régulation en tension avec boucle d'anticipation dite "Feed-Forward".
Régulation en tension simple
La génération de rampe pour le mode de régulation en tension "simple" est assurée par la résistance R14. Cette résistance de 0Ω assure le bouclage entre les broches 'Ct' et 'Ramp' du contrôleur, voir les datasheets du contrôleur [4], [5], [6] ou [7].
Régulation en courant
Le signal rampe nécessaire au mode de régulation en courant est prélevé en sortie du filtre formé par la résistance R16 et le condensateur C14, voir les datasheets du contrôleur [4], [5], [6] ou [7].
Régulation en tension avec Feed-Forward
Ce mode de régulation nécessite de générer un signal en dent de scie périodique, dont l’amplitude évolue en fonction de la tension d’alimentation, comme illustré en figure 4 (pour plus de détails voir [2], [8] et [9]).
La génération du signal rampe est assurée par le schéma de la figure 5 conçu sur la base du schéma de principe proposé par le constructeur (voir les datasheets [4], [5], [6] ou [7]) et des théories exposées par les documents [8] et [9].
La lecture (de gauche à droite) du schéma de la figure 5 (dont le principe est illustré en figure 6) montre que le signal rampe est généré aux bornes du condensateur C17, qui se charge à travers la résistance R20 et se décharge à l’aide de la résistance R24 lorsque le transistor Q3 est passant. Ce transistor est commandé par le signal CLK dont la composante continue (2.3V environ) est supprimée par le filtre formé de la résistance R26 et du condensateur C16. Cependant suivant la valeur du condensateur C16, le signal CLK nécessite d’être amplifié en courant, ce qui est réalisé par le circuit suiveur formé du transistor Q4 et des résistances R22 et R25. Cette dernière assure aussi un rôle lors de la décharge de C16 lorsque le signal CLK retourne au niveau bas. Enfin l’amplitude de la rampe est limitée à environ 6V à l’aide de la diode D7.
La résistance R27 de valeur 0Ω offre la possibilité d’isoler la structure pour en faciliter la mise au point.
Les valeurs des composants sont estimées de la manière suivante.
Dans un premier temps les valeurs de R24 et C17 doivent permettre de décharger complètement ce condensateur durant l’état haut du signal CLK, soit pendant la période de temps mort ‘td’. Cette exigence impose le critère ci-dessous.
R24 * C17= td / 20
Soit.
C17≤ (td / 20) * R24
Ensuite, la valeur de C17 précédemment calculée permet une estimation de la résistance R20, en fonction de la tension VFF, de la tension RAMP désirée et des périodes de découpage TCLK et de temps mort ‘td’.
R20= VFF / (20 * Irampe)
Avec
Irampe=( Vrampepk * C17) / (TCLK - td)
Enfin le condensateur C16 et la résistance R26 sont calculés pour assurer la conduction du transistor Q3 pendant la période de temps mort ‘td’. Ces éléments sont estimés sur la base des équations suivantes.
C16 ≤ 1e-3 * td
R26 * C16 = 5 * td
Points de test
Les points de test ont pour utilité de permettre la mesure des signaux du contrôleur sans les perturber.
Amplificateur d'erreur / Correcteur
Cet étage est sans doute le plus important, puisqu'il est la clé d'une bonne régulation de l'alimentation. Ce circuit est basé sur un bloc préconçue pouvant être configurée soit en correcteur de type 1, 2, 3 ou en amplificateur suiveur en fonction du besoin de l'application.
L'étude et la conception de l'amplificateur d'erreur est basé sur les ouvrages [1] ou [2].
Les différentes configurations sont les suivantes.
- Si l’amplificateur interne au contrôleur est utilisé en suiveur, dans ce cas les résistances R2 et R11 sont montées, le condensateur C10 et remplacé par une résistance de 0Ω et la valeur de la résistance R7 est adaptée en fonction du besoin.
- Si l’amplificateur interne au contrôleur est utilisé en correcteur de type 1, 2 ou 3, alors la résistance R11 n’est pas montée et les valeurs des autres éléments dépendent du type de correcteur à mettre en œuvre, ainsi que des estimations.
Ce paragraphe conclut le volet conception de la carte, passons à la réalisation pratique.
Réalisation pratique
La réalisation pratique d’une telle carte n’est pas une chose aisée, car elle repose en majeur partie sur l’expérience du concepteur, qui ne s’acquiert que par la pratique, j’ai donc naturellement débuté par la réalisation des maquettes sur cartes pastillées. Ces maquettes m’ont permis de me lancer dans la mise au point et la vérification de la faisabilité sur des prototypes que je pouvais « re-router » au fer à souder. Au terme de trois versions de prototypes « labo », je suis arrivé à la quatrième version qui a été routée, voir le PCB en figure 9 et 10. La carte se présente sous un format de 50mm x 50mm, donc très chargé vu le nombre de fonctions à implémenter. Le contrôleur MA1 est monté sur un support DIP16 pour faciliter le remplacement en cas de dégradation.
Le connecteur J1 peut être au choix soit une barrette sécable droite ou coudée.
Après fabrication des circuits, il suffit simplement de configurer les différentes fonctions pour se lancer dans une première application.
Application
Lors de la mise au point des premières cartes prototypes, j’ai réalisé, deux types d’alimentation à découpage, une Buck et une Forward (voir photos 1, 2 & 3). J’ai donc bien sûr réutilisé les cartes puissances de ces alimentations pour mener la mise au point et les premiers essais, afin de comparer les performances entre les cartes prototypes pastillées et les cartes routées.
Evolutions / Améliorations
Amélioration Over-Voltage LockOut
Au moment de boucler cet article, j’ajoutais l’amélioration décrite ci-dessous.
En effet, la conception de base du circuit Over-Voltage LockOut ne permet pas de
programmer un seuil de déclenchement très précis. Ce défaut est principalement dû à la variation de la tension Vbe du transitor Q5. Cette valeur pouvant varier entre 0.5V et 1V, il en résulte que la valeur du seuil, calculée à l'aide de l'équation ci-dessous, peut varier du simple au double (soit une erreur de 100%).
SeuilOVLO = VbeQ5 * (R28+R29)/R29
Ce problème peut être résolu par l'ajout du petit montage proposé en figure 7. Ce schéma, permet d’activer le transistor Q5 (donc le système Over-Voltage LockOut) lorsque le transistor Q6 est passant, quand la tension aux bornes de la résistance R31 dépasse la valeur de VREF+VbeQ6 (soit entre 5.6V et 6.1V environ). Ce principe permet de réduire l'erreur de la tension de seuil du système Over-Voltage LockOut à une valeur de 5.85V +/-5%.
Ainsi l'équation de calcul du seuil devient la suivante.
SeuilOVLO = (VREF + VbeQ6) * (R31+R32)/R31
Pour cela, les valeurs des composants sont :
Q6 = MMBT2907 (SOT23)
R29 = 10k (0805)
R30 = 100k (0805)
R31 = 10k (0805) à ajuster si besoin
La résistance R32 est calculée à l’aide de l’équation ci-dessous et du seuil de déclenchement désiré.
R32 = [SeuilOVLO - (VREF + VbeQ6)] / [(VREF + VbeQ6)/R31]
Nota : dans le cas de l’utilisation de ce circuit, R28 n’est pas connectée.
En conclusion cette amélioration réduit sensiblement l’erreur de seuil du circuit Over-
Voltage LockOut et sera implémentée sur la prochaine version de PCB. Néanmoins les photos 2 et 3 laissent figurer un exemple d’implémentation, pour ceux d’entre vous désirant utiliser cette amélioration sur la version 04 du PCB.
Conclusion
Les résultats obtenus avec les cartes routées sont très proches de ceux obtenus avec les cartes prototypes pastillées, ce qui démontre, malgré leur réputation, que les alimentations à découpage à base de composant discrets sont accessibles même aux amateurs, pour peu que le câblage soit soigné et si l’on met de
côté l’aspect contraignant de la CEM.
Liens
[1] Switch-Mode Power Supplies Spice Simulations and Practical Designs, C Basso, 2010, ISBN-13 : 978-8183332910
[2] Switching Power Supply A to Z, S. Maniktala, 2006, ISBN-13 : 978-0750679701
[3] Power Supply Cookbook, Second Edition, M. Brown, 2001, ISBN-13 : 978-0750673297
[4] Datasheets UC2823 : http://www.ti.com/lit/ds/symlink/uc2823.pdf
[5] Datasheets UC2824 : http://www.ti.com/lit/ds/symlink/uc2824.pdf
[6] Datasheets UC2825 : http://www.ti.com/lit/ds/symlink/uc2825.pdf
[7] Datasheets UC28025 : http://www.ti.com/lit/ds/symlink/uc28025.pdf
[8] Datasheets CS51221 : http://www.onsemi.com/pub_link/Collateral/CS51221-D.pdf
[9] Datasheets LM25037 : http://www.ti.com/lit/ds/symlink/lm25037.pdf
Discussion (2 comments)
ClemensValens 9 years ago
Dear Mikaël,
Can you please add component values to the schematics or a bill of materials. I know some part values are experimental, but others are not.
Regards,
Clemens
Mickael.Delayen 8 years ago
Mickael.Delayen 8 years ago
Regards,
Mickaël
ClemensValens 10 years ago
Dear Mikaël,
Thank you for your interesting proposal, but can you please explain who is supposed to use this controller board? You don't explain how to use it, there is no power stage and it is a rather costly solution that requires two PCBs. Except for the controller chip it is all SMD, placed on both sides, that makes it hard to use in educational applications. So?
Best regards,
Clemens
Mickael.Delayen 10 years ago