Team Observe Quantum Effects in Fe Superconductors
November 25, 2019
on
on
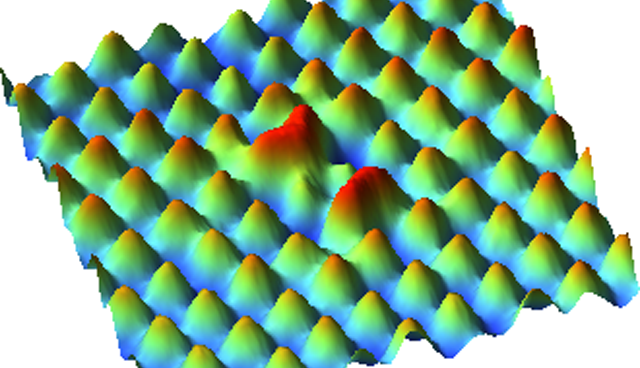
Superconductors allow electricity to flow without encountering electrical resistance. More recent iron-based alloys remain superconducting even at relatively high temperatures (for super conductors). How superconductivity actually works in iron-based materials is still a mystery, since the magnetic properties of iron should hinder superconductivity.
Researchers at Princeton University studied superconductivity in iron-based alloys which had varying concentrations of cobalt added. How superconductivity arises in iron-based alloys is something of a conundrum because iron's magnetic properties should confound the emergence of superconductivity. According to Anderson's Theorem of 1959, additional impurities in the alloy will interfere with superconductivity but in many cases will not destroy it completely. Cobalt is an exception; when it’s added to iron-based superconductors, superconductivity is lost; the reason for this was unclear.
The team used a scanning tunneling microscope to examine a large number of samples (at a temperature of around 400 degrees milliKelvin) containing varying concentrations of cobalt impurities. The influence of cobalt atoms on superconductivity could be measured at both an atomic and global level. It turned out that every cobalt atom exerts a limited local influence that diminishes one or two atoms away from the impurity site. When the cobalt concentration increases, the alloy’s superconducting property disappears completely.
Super conducting quantum-phase effect. Video: Princeton University.
Superconductivity arises from the coupling of two electrons to make a single quantum state. This allows electrons to pass through a material without encountering resistance. For lithium iron arsenide, scattering at the so-called Born boundary is apparently capable of violating Anderson's theorem, resulting in a quantum phase transition from a superconducting to a non-superconductive state.
Superconductors can also be described by a property called their ‘tunneling spectrum’. Lithium iron arsenide has an 'S-wave gap' with U-shaped bottom in the superconducting energy gap. The cobalt impurities not only suppress superconductivity, they also change the nature of the gap. The team found that by introducing a sign change in the superconductivity order parameter they were able to replicate the odd changes produced by the cobalt impurities. Their conclusion, backed up by three theoretical models, indicated that the superconductor was indeed a sign-changing exotic alloy not covered by Anderson’s original work.
The findings were published in Physical Review Letters.
Researchers at Princeton University studied superconductivity in iron-based alloys which had varying concentrations of cobalt added. How superconductivity arises in iron-based alloys is something of a conundrum because iron's magnetic properties should confound the emergence of superconductivity. According to Anderson's Theorem of 1959, additional impurities in the alloy will interfere with superconductivity but in many cases will not destroy it completely. Cobalt is an exception; when it’s added to iron-based superconductors, superconductivity is lost; the reason for this was unclear.
The team used a scanning tunneling microscope to examine a large number of samples (at a temperature of around 400 degrees milliKelvin) containing varying concentrations of cobalt impurities. The influence of cobalt atoms on superconductivity could be measured at both an atomic and global level. It turned out that every cobalt atom exerts a limited local influence that diminishes one or two atoms away from the impurity site. When the cobalt concentration increases, the alloy’s superconducting property disappears completely.
Super conducting quantum-phase effect. Video: Princeton University.
Superconductivity arises from the coupling of two electrons to make a single quantum state. This allows electrons to pass through a material without encountering resistance. For lithium iron arsenide, scattering at the so-called Born boundary is apparently capable of violating Anderson's theorem, resulting in a quantum phase transition from a superconducting to a non-superconductive state.
Superconductors can also be described by a property called their ‘tunneling spectrum’. Lithium iron arsenide has an 'S-wave gap' with U-shaped bottom in the superconducting energy gap. The cobalt impurities not only suppress superconductivity, they also change the nature of the gap. The team found that by introducing a sign change in the superconductivity order parameter they were able to replicate the odd changes produced by the cobalt impurities. Their conclusion, backed up by three theoretical models, indicated that the superconductor was indeed a sign-changing exotic alloy not covered by Anderson’s original work.
The findings were published in Physical Review Letters.
Read full article
Hide full article
Discussion (0 comments)